MAY THE FUSION BE WITH US!
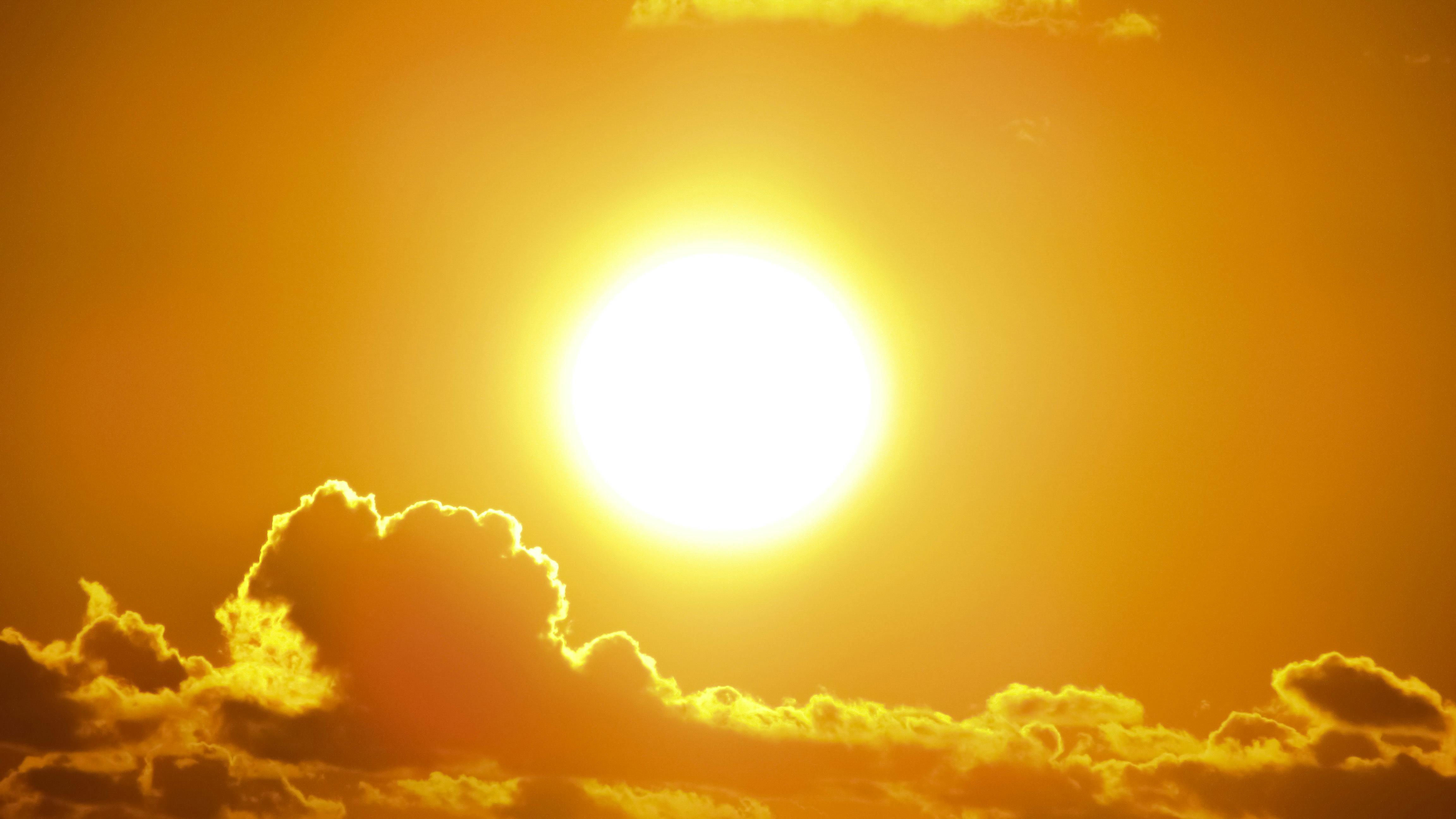
Energy is the fundamental currency for our universe. It lights our cities, grows our food and runs our machines. Humans, for thousands of years were able to get this from different means. By burning fossil fuels, splitting atoms by Nuclear fission and photovoltaic cells exposed to sunlight. But then again everything has a dark side to it. Burning fossil fuel produces poisonous gases, atomic energy waste are radioactive and we are still not able to find means to capture solar energy in low intensity regions or in cloudy days.
Then when we look up to our Sun it seems to have virtually unlimited supply of energy. Is there a way we can house a sun on planet earth or by any means we can bottle a star?
Stellar evolution says the lifetime of a star can range from a few million for the most massive to trillions of years for the least massive, which is considerably longer than the age of the universe.
Ever wondered how these twinklers in our night sky are able to live for such long time?
The sun and all the other stars in the universe are powered by Nuclear fusion. This process continuously goes on at the Sun's core. In a nutshell nuclear fusion can be defined as a thermonuclear process. This means the elements have to be so hot that the electrons are ripped off the atoms (get ionized) to form the most energetic state of matter - Plasma. In this state the nuclei are free to interact with each other. Hence, the lighter nuclei especially that of hydrogen fuse together to produce heavier nuclei- Helium nuclei (as per the Nuclear binding energy curve). Since the heavier nuclei produced are more tightly bound than the lighter nuclei there is a release of energy. This energy powers every star out in the night sky and our Sun.
But the nuclei are of like charge, so they will repel each other when brought close. To have high probability in occurrence of Nuclear fusion the nuclei need to go very fast or have large kinetic energy. As per this context having high kinetic energy means the temperature of Sun's core must be very high like millions of degrees. So high that the nuclei gain enough energy to overcome the coulomb barrier to undergo fusion reaction.
The solar core has temperature of 15 million kelvins (15 million degrees Celsius, 27 million degrees Fahrenheit) and a density of 150 g/cm^3 (150 times the density of liquid water). The core is very dense and the pressure (estimated as 265 billion bar), which squeezes the matter close enough to achieve temperature range for fusion reaction. But studies show the temperature of Sun's core not that sufficient to cause fusion reaction. As per quantum physics, the phenomenon of nuclear fusion in stars can be explained through the quantum tunneling effect. It is a phenomenon where a subatomic particle disappears from one side of the potential barrier and appears on the other side without any probability of appearing inside the potential well even though it doesn't have enough energy. Thus, Quantum tunneling increases the probability of penetration of nuclei through the coulomb barrier. Though this probability is low, the large density of nuclei in core of a star compensates it and hence stars are able to sustain a steady fusion reaction releasing energy for millions, billions, or even trillions of years.

FUSION POWER

The Joint European Torus (JET) magnetic fusion experiment in 1991
Nuclear fusion power is the holy grail of energy for the future. It has potential for becoming virtually limitless source of clean energy. It is this release of energy that scientists hope to harness in a new generation of power plant using devices designed to generate electricity from this energy, known as fusion reactors . On earth it is not possible to use forces that cause fusion in stars. In the star their gravity helps to confine the plasma long enough to reach the condition of nuclear fusion producing large amount of energy. For power generation purposes on earth we need a engineered reactor with fuel and a confined environment with sufficient temperature, pressure and confinement time to sustain a plasma for fusion reaction to occur.

Lawson criterion:
Combination all these factors essential for producing fusion power sums up to the Lawson criterion. It shows the relation between the energy output value and the temperature, pressure ,speed of collision and fuel. This equation was central to John Lawson's analysis of fusion working with a hot plasma. The energy balance is shown below :-
Plasma lose energy through conduction and radiation. Conduction occurs when particles like the electrons, ions and neutrons collide with the device's plasma facing wall and transfer their kinetic energy as heat to the wall. Radiation is energy that leaves the cloud as light in the visible, UV, IR, or X-ray spectra. In any fusion reactor these loses have to be taken care of for achieving higher efficiencies. The argument provided by the Lawson criterion is that a machine operating with a thermalized and quasi-neutral plasma has to meet basic conditions to overcome radiation losses, conduction losses, and reach efficiency of about 30 %.
Energy extraction:
Multiple methods have been experimented for the capture of heat energy from a fusion reactor and generating electricity from it. Most convincing way is to heat working fluid (sub-cooled water). Most reactors use Deuterium-tritium reactions which releases most energy in form of kinetic energy of product neutrons. Neutrons are electrically neutral, hence escape the magnetic confinement. In such designs subsequently they are captured by the thick blanket of lithium surrounding the reactor core. When struck by a high-energy neutron, the lithium can produce tritium, which is then put back into the reactor. The energy of this reaction also heats the blanket, which is then actively cooled by pressurized sub-cooled water and then the fluid is used to drive conventional turbo-machinery for generating electricity.
There has been proposed plans to use the neutrons from nuclear fusion to breed additional fission fuels in a blanket where waste products are form in a nuclear fusion reactor. This concept is known as Fission-Fusion hybrid. Power production will be enhanced by integrating fusion and fission reactions. The heat from the hybrid reactor is extracted through conventional methods by using turbomachineries.
Designs that use other fuels, notably the proton-Boron reaction, release much more of their energy in the form of charged particles. In these cases, alternate power extraction systems based on the movement of these charges are possible. Magneto-Hydrodynamics can also be used for energy conversion from heat to electricity.
Examples of magnetofluids include plasmas, liquid metals, salt water, and electrolytes. The principle behind MHD is that magnetic fields can induce currents in a conductive fluid in motion, which provides a feedback by polarizing the MHD fluid and reciprocally changes the magnetic field itself.
Confinement:
Fusion researchers have investigated various confinement concepts. Till date scientist have been successful in experimenting with two main kinds to confinement technologies, Magnetic Confinement (Tokamak and stellarators ) and Inertial Confinement using high energy Lasers. Both designs are under research at very large scales, most notably the ITER tokamak in France, and the National Ignition Facility laser in the United States. Engineers and scientists are also testing other alternatives for feasible fusion reactors, among them are magnetized target fusion and inertial electrostatic confinement, and new variations of the stellarator.
- Magnetic confinement fusion is an method for generating thermonuclear fusion power that uses strong magnetic fields produced by superconducting magnets to confine fusion fuel in the form of a high temperature plasma. Magnetic confinement fusion attempts to use the electrical conductivity of the plasma to contain it with magnetic fields. The major experimental reactors such as Tokamak and stellarators use magnetic confinement. Usually plasma is confined in a toroidal (doughnut) shaped configuration. (Image below)

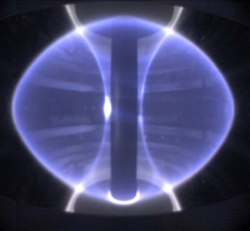
Fig 1 & 2: Magnetic Confinement of Plasma
- Inertial confinement fusion (ICF) is a type of fusion energy and reactor research that attempts to initiate fusion reactions by heating and compressing a fuel pellet that most often contains a mixture of deuterium and tritium. To compress and heat the fuel, energy is delivered to the outer layer of the target using high-energy beams of laser. Then due to intense heating the reaction occurs and the outer layer of pellet explodes outwards providing an reaction force to the inner fuel materials, compressing the target. For this method the reactor is designed such that the shock waves created by explosion of outer layer of pellet can induce fusion reaction at the core.
Fig 3: Inertial confinement with lasers.
Fuel:
1)Deuterium-Tritium :-

The most common reaction used in present generation nuclear fusion reactors for experimental purposes in Deuterium-Tritium reaction. Usually used as a convenient source of neutrons. Deuterium is a naturally occurring isotope of hydrogen, which is abundantly found in sea water. The large mass ratio of hydrogen isotopes makes it easier for extraction from natural sea water as compared to the uranium enrichment process in nuclear fission reactors.
Tritium is a natural isotope of hydrogen and very hard to find, store and produce. Consequently, the deuterium-tritium fuel cycle requires the breeding of tritium from lithium using one of the following reactions:
At least some neutron multiplication reactions are required to compensate for the neutrons lost to absorption by other elements. Leading candidate neutron multiplication materials are beryllium and lead however the Lithium-7 reaction above also helps to keep the neutron population high. Most reactor designs use breeder blankets with enriched Li-6.
Several drawbacks are commonly attributed to D-T fusion power:
- It produces a sufficient number of neutrons that result in the neutron activation of the reactor materials.
- Only about 20% of the fusion energy yield appears in the form of charged particles with the remainder carried off by neutrons, which limits the application of direct energy conversion techniques.
- It requires the careful handling of the radioisotope tritium. Similar to hydrogen, tritium is difficult to contain and may leak from reactors in some quantity.
2) Deuterium - Helium-3 :-
A second-generation approach to controlled fusion power involves combining helium-3 and deuterium:
Similar to the p-B aneutronic fusion fuel cycle, most of the reaction energy is released as charged particles, reducing activation of the reactor housing and potentially allowing more efficient energy harvesting as compared to conventional use of turbo-machinery. The Charged particles released with a imparted momentum can then be used to produce current in a properly configured conductor.
3)Proton, boron-11:-
The most promising candidate fuel for aneutronic fusion is fusing the readily available hydrogen-1(i.e. a proton) and boron. Their fusion releases no neutrons, but produces energetic charged alpha (helium) particles whose energy can directly be converted to electrical power:
Because the confinement properties of conventional approaches to fusion such as the tokamak and laser pellet fusion are marginal, most proposals for aneutronic fusion are based on radically different confinement concepts, such as the Polywell and the Dense Plasma Focus. In 2013 a research at École Polytechnique in Palaiseau, France, reported a new fusion rate record for proton-boron fusion, with an estimated 80 million fusion reactions during 1.5 nanoseconds laser fire, over 100 times more than previous proton-boron experiments.
Future prospects:
If successfully commercialized, fusion power will generate more energy for a given amount of fuel than any other conventional fuels like fission elements and fossil fuels that are currently in use.
In a nutshell, it will be so efficient that a single glass of sea water can produce energy as much as a barrel of oil with absolutely no considerable waste.
The fuel itself (primarily deuterium) exists abundantly in the Earth's ocean: about 1 in 6500 hydrogen atoms in seawater is deuterium. It may seem less but the fusion reactions are more energetic than other reactions, and seawater is easier to access and more plentiful than fossil fuels, fusion could potentially supply the world's energy needs for millions of years. But still for some a fusion reactor may still sound dangerous. In reality it will be the most safer kind of power plants in terms of fatalities than others. Let's compare it with nuclear fission energy the safest energy till now on earth, a fusion reactor is not like a fission power plant which can meltdown catastrophically release harmful radiations. If the confinement in a nuclear fusion reactor fails the plasma will expand and cool down, and the reaction would stop. Hence, nuclear fusion reactors are not bombs.
There is one minute problem with the radioactive isotope of hydrogen used, tritium. If the tritium spills out it could react with oxygen to form toxic, radioactive water and if it flows into water bodies it could pose a threat to the ecosystem. But, fortunately only a few grams of tritium is released and when it mixes with normal water it will be quickly diluted. Thus, fusion energy will be a apparently unlimited source of energy for our world without any harm to the environment, from a fuel obtained from seawater which is a renewable source( water cycle ).
As per studies Helium-3, an isotope of helium can act as an alternative for tritium in a fusion reactor. But it is available in so scarce amount on earth that it would have to be mined extra-terrestrially or produced by other nuclear reactions. Our natural satellite the moon seems to have a large deposit of this Helium-3 isotope as the moon dust. Due to years of interaction of lunar soil with the solar radiations the soil was able to convert into deposits of Helium-3.
Challenges:
The fact that we don't have Fusion power today is because it takes billions of dollars to build a reactor and it always seem to be perpetually 30 years away from a viable nuclear fusion power plant. We still have no idea that Fusion power will ever get economically viable. It's technology is still unproven, every reactor at this moment around the world are merely experimental. The appropriate combination of temperature, pressure and duration of confinement has proven to be difficult to produce fusion power in a practical and economical manner. Research into fusion reactors began in the 1940s, but to date, no design has produced more fusion power output than the electrical power input, defeating the purpose.
Just to show how costly it can be let's take the instance of ITER. The project is funded and run by seven member—the European Union, India, Japan, China, Russia, South Korea and the United States. The EU, as host party for the ITER complex, is contributing about 45 percent of the cost, with the other six parties contributing approximately 9 percent each. Construction of the ITER Tokamak complex started in 2013 and the building costs were over US$14 billion by June 2015.
Apart from the cost there is another issue with the fusion reactor is the management of neutronic loads, neutrons that are released during the reaction, which over time degrade many common materials used within the reaction chamber. But we can use materials in the blanket wall which when react with the neutrons can transmutate into other usable fuel materials.
But it's worth spending resources on Fusion power for achieving sustainable development.
0 Comments