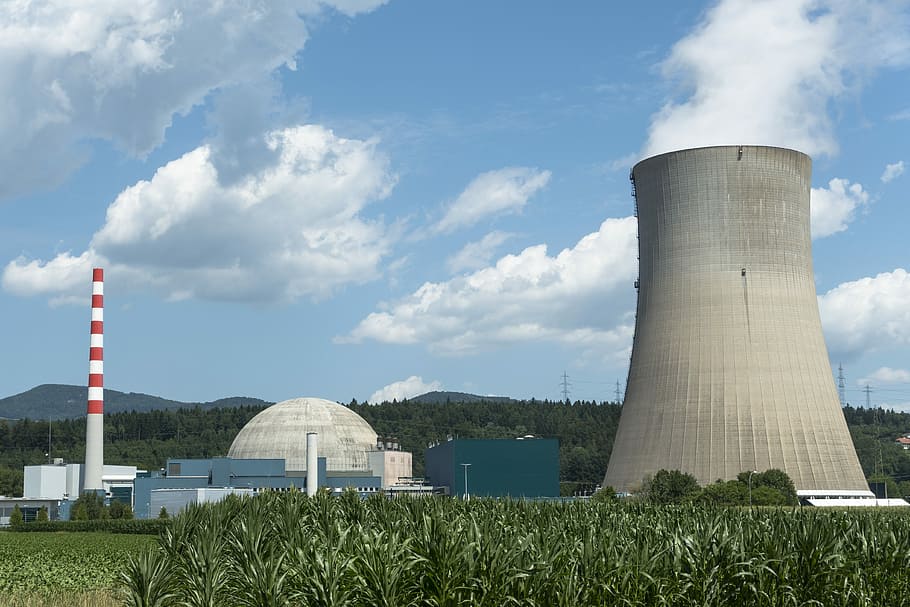
Isn't our viewpoint on availability of energy quite ambiguous? Basically, energy we need is not scarce. It is literally everywhere and in everything. As in the previous post on nuclear energy we discussed that mass is after all just another form of energy. Then what we really mean when we say Energy is the solution for all sufferings of our world? If there is energy everywhere why go for solutions? The trick is to get that energy available around us in a way that presents minimal threat to environment and human life. We burn coal and liberate a small amount of its energy locked in its chemical bonds. It's easy and economical but the energy release is pathetic for a kilogram of coal burned and worse for a ton of carbon dioxide released to the atmosphere. At the other end of the spectrum is the energy we get by splitting atoms in nuclear power plant. Until now is the only viable option of nuclear power is by Nuclear fission. For capturing such energy from fission we need sophisticated and robust engineered devices - known as Nuclear Reactors.
Nuclear reactors:-
Nuclear reactors are a class of devices, formerly known as an atomic pile, that can initiate and control a self-sustaining nuclear fission reaction - Nuclear chain reaction. Mostly used in power plants for electricity generation, as research reactors, system for breeding of radioactive isotopes or for marine propulsion. Heat extracted form a nuclear reactor is transferred to a working fluid - water, gas or molten salt, which in turns drives steam turbines. These turbines are then used to either drive a ship's propellers or turn electrical generators' shafts. As of early 2019, the IAEA reports there are 454 nuclear power reactors and 226 nuclear research reactors in operation around the world.
Operation of a nuclear reactor:-
Nuclear reactors work on the principle of Nuclear fission, the process in which heavy atomic nuclei are split into two lighter fragments. The nuclear fragments are in very excited states and emit neutrons, other subatomic particles, and photons.
Notice one fact of great significance in the fission reaction given above. There is release of extra neutrons in the process. Averagely, two and half neutrons are released from fission of uranium nucleus. It is a fraction because in some there are 2 neutrons and in some 3. The extra neutrons in turn can initiate more fission reactions, producing still more free neutrons. This can leads to a chain reaction, as was first suggested by Italian physicist Enrico Fermi, the creator of the world's first nuclear reactor, the Chicago Pile-1. A large amount of energy is released in this process, and this energy is the basis of nuclear power systems. If the chain reaction is controlled suitably, we can get a steady energy output.
In an atomic bomb, the same nuclear fission occurs but is designed such that it's intensity increases until much of the material has fissioned. This process occurs very rapidly and causes a tremendously energetic explosion. Basically, it is an uncontrolled chain reaction. In a nuclear reactor the chain reaction is maintained at a controlled, nearly constant level. Nuclear reactors are so designed that they cannot explode like atomic bombs.
Most of the energy (approximately 85 percent of it) from nuclear fission is liberated during the short interval of occurrence of the reaction. The rest is produced as a result of radioactive decay of of fission products, which are fission fragments after they have emitted neutrons. By this the radioactive nuclei reach a stable state. The decay process continues even after fissioning has ceased, and its energy must be dealt with in any proper reactor design.
Figure:- Cherenkov radiation in a nuclear reactor.
Slow and steady :-
Achievement of chain reaction highly depends on the probability (cross-section) that the neutrons released in a particular fission reaction can cause subsequent fission. Mainly there are two kinds of neutrons according to their energy range, Thermal (in eV) and fast (in MeV) neutrons. It is known experimentally that slow neutrons (thermal neutrons) are much more likely to cause fission in U-235 than fast neutrons. U-235 is the most abundant highly fissile isotope of uranium used in most commercial Nuclear power plants. Fast neutrons can also be used in nuclear reactors, so called fast reactors.

Cross-section of U-235 vs Energy
The average energy of a neutron released in fission of U-235 is 2 MeV. Before they are slowed down by a large number of nuclear collisions, neutrons produced by fission reactions are considered fast. The substantial amount of kinetic energy in these fast neutron allows them to fission more nuclei once they get captured. But cross section of capture (probability of getting captured by the medium nuclei) for these fast neutrons are small as compared to the slow neutrons (graph above). Unless slowed down these neutrons will escape from the core without interacting with fuel nuclei. As a result, an intense neutron flux and a fuel rich in fissile elements are both needed to compensate for this lower probability. What one needs to do is to slow down the fast neutrons by elastic scattering with light nuclei.
Slowing down of neutrons | Moderator :-
Figure:- N - Average number of collisions of a neutron to nucleus to slow down from E1 = 1 MeV to E2 = 1 eV
In fact, Chadwick's experiments showed that in an elastic scattering with hydrogen the neutron almost comes to rest and proton carries away the energy. This is the same situation as when a marble hits head-on an identical marble at rest. Thus, water along with the fuel rods in a reactor core can be used as moderators for slowing down fast neutrons. In doing so, the moderator helps initiate and sustain a fission chain reaction.
Figure:- Table showing slowing down power and Moderation ratio for different moderators.
Some nuclei have larger absorption cross sections than others, which reduce free neutrons from the flux. Therefore, a further criterion for an efficient moderator is one for which absorption parameter is small. The moderating efficiency gives the ratio of the macroscopic cross sections of scattering, weighted by average logarithmic loss in energy for mixture of nuclei, divided by that of absorption cross-section (last column of above table). Therefore, neutrons are more rapidly moderated by light water, as H has a far higher scattering cross-section. However, it also has a far higher absorption cross-section, so that the moderating efficiency is far higher for heavy water than for light water. "Light water" is the most commonly used moderator (roughly 75% of the world's reactors). Solid graphite (20% of reactors) and heavy water (5% of reactors) are the main alternatives.
The ideal moderator is of low mass, high scattering cross section, and low absorption cross section.
Chain reaction and criticality:-
The ratio K, known as multiplication factor, defined as the ratio of fission produced by a given generation of neutrons to the number of fission of the preceeding generation. It is an important factor for a self sustaining nuclear reactor. It is a measure of growth rate of neutrons in a reactor.
- If the number of neutrons produced decreases for subsequent reactions, the rate of fission will decrease ultimately dropping to zero. This state of the reactor is known as sub-critical state (K<1).
- If over the course of time the neutron population is sustained at a constant rate, the fission rate will remain steady, and the reactor will be in what is called a critical state (K=1).
- If the neutron population increases over time, the fission rate and power will increase exponentially, and the reactor will be in a supercritical state (K>1). Unless by any means K is brought close to unity, the reactor can explode and cause catastrophic consequences.
Reaction Control :-

The reactivity of a nuclear reactor is controlled by control-rods. Reactivity is positive when a reactor is supercritical, zero at criticality, and negative when the reactor is subcritical. These rods (figure above) are composed of non-fissile chemical elements like boron, cadmium, silver, or indium, which are capable of absorbing neutrons, hence reducing the neutron flux for achieving criticality. Each reactor design can use different control rod materials with appropriate absorption cross-section based on the energy spectrum of its neutrons. Before start-up the population of neutrons is zero. During start-up these rods are removed so that reaction can occur, effectively putting the reactor temporarily into a supercritical state. For a steady state operation the control rods are partially inserted to bring down the number of neutrons to a nominal state. And for shutting down a reactor the rods are fully inserted to halt the fission process by reducing the free neutrons.
Changes of neutron leakage, on the other hand, are often automatic. Increase in number of neutrons will increase power output, this increase in power will tend to decrease the coolant density and probably would boil. This decrease in coolant density will increase neutron leakage out of the system and thus reduce reactivity, a process known as negative-reactivity feedback.
Fuel enrichment :-
Reactors fuels must conform with the design and operation of a nuclear reactor. Most of the 500 commercial nuclear power reactors operating or under construction in the world today require uranium 'enriched' in the U-235 isotope for their fuel. Naturally found uranium consist of two isotopes :- U-235 and U-238. The production of energy in nuclear reactor is through the fission of U-235. U-235 is the main fissile isotope of uranium. Natural uranium contains 0.7% of the U-235 isotope. The remaining 99.3% is mostly the U-238 isotope which does not contribute directly to the fission process (though it does so indirectly by the formation of fissile isotopes of plutonium). Isotope separation is a physical process to concentrate (‘enrich’) one isotope relative to others. Most reactors are light water reactors (of two types – PWR and BWR) and require uranium to be enriched from 0.7% to 3-5% U-235 in their fuel.
The light water reactor (LWR) is the most widely used nuclear reactor for power generation purposes. These reactors employs a fuel consisting of pellets of sintered uranium dioxide loaded into cladding tubes of zirconium alloy or some other advanced cladding material. Since, in LWRs water acts as both as a moderator and coolant, as seen earlier it has high absorption cross-section, tends to absorb more neutrons than other moderators do, such enrichment is crucial. The moderation efficiency of heavy water is large as compared to light water. So, heavy water reactors use natural uranium compacted into pellets, these pellets are inserted in long tubes and arranged in a lattice.
Cooling and Power generation :-
Figure:- Schematic diagram of nuclear reactor based on thermal neutron fission.
The core is the site of nuclear fission. It contains fuel elements in suitably fabricated form. The core contains a moderator to slow down neutrons. At the moment of the reaction a significant portion of the fission energy is converted to heat. This heat energy deposited in the core of a reactor and a coolant is required to remove the heat in order to maintain a balanced system (and also to transfer the heat energy to the power-generating plant). The most common coolant is water, though any fluid can be used. Heavy water (deuterium oxide), air, carbon dioxide, helium, liquid sodium, sodium-potassium alloy (called NaK), molten salts, and hydrocarbons have all been used in reactors or reactor experiments. For high power nuclear reactors we need reliable and sophisticated cooling systems to extract heat effectively, otherwise the heat will gradually build up and the reactor can melt down. Systems that enable sufficient cooling during all credible abnormal conditions in nuclear power plants are referred to as emergency core-cooling systems.
In power producing reactors we need to remove as much as heat possible for generating useful power, generally electricity out of it. For this a coolant fluid enters at low temperature and exits at high temperature after capturing heat from the core. In most light-water, heavy-water, and gas-cooled power reactors, the coolant is maintained at high pressure. Sodium and organic coolants operate at atmospheric pressure. Then this high temperature coolant is used to produce steam, which in turn drives a conventional steam turbine. Electricity is produced by connecting this turbine shaft to a generator. Then the steam is converted to liquid water and cycled back to the steam producing unit hence completing the cycle. This thermodynamic cycle is known as Rankine cycle. Cooling towers (the massive chimneys you see around any nuclear power station ) are used to liquefy the steam after passing it over the turbine. Not all nuclear power plants use cooling towers, some instead cool their heat exchangers with lake, river or ocean water. Discussed above is called a pressurised water reactors (PWR). In a boiling water reactors (BWR) the coolant usually water, is directly converted to steam inside the reactor by boiling it. This is known as pool boiling. More on different types of nuclear reactors in upcoming posts.
Shielding and Containment:-
Any operating nuclear reactor is a source of harmful radiations. Reactors are designed with the expectation that they will operate safely without releasing radioactivity to their surroundings. Fission and subsequent radioactive decay produce neutrons and gamma rays, both of which are highly penetrating radiations. The design of a reactor must be appropriate with barriers for absorbing and reflecting this radiation in order to protect reactor personnel from exposure. These barriers are, the fuel cladding, the reactor vessel, and the shielding. As a final barrier, the reactor is housed in a containment structure. The shielding is provided by placing the reactor in a large, deep pool of water. In other kinds of reactors, the shield consists of a thick concrete structure around the reactor system referred to as the biological shield. The shield also may contain heavy metals, such as lead or steel, for more effective absorption of gamma rays, and heavy aggregates may be used in the concrete itself for the same purpose. The containment usually consist of a cylindrical reactor building with a spherical dome. The containment structure must be at least nominally airtight. It has to withstand pressure buildups and damage from debris propelled by an energy burst within the reactor. They also must safeguard the reactor components inside it from natural calamities.
0 Comments